Re Research by Hitoshi Okamoto and his colleagues
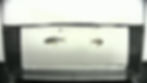
How has the brain evolved to accommodate our "mind"?
~the history and aims of my research~
We originally studied the specification of motor neurons in terms of their position, shape, and axonal trajectory. In particular, by using our original transgenic zebrafish line that expresses GFP in motor neurons both in the hindbrain and the spinal cord for the forward saturation mutagenesis screening, we showed that a group of genes regulating the planar polarity and the apicobasal polarity of the neuroepithelial cells is involved in the correct positioning of the hindbrain cranial motor neurons.
In parallel with this genetic study of motor neuron differentiation, we accidentally discovered that the neurons in the medial and lateral subnuclei of the dorsal habenula show topographic projections to the ventral and dorsal interpeduncular nucleus (IPN) as different motor neurons project topographically to different muscles. Unlike neuro-muscular projections, the functions of the habenula-IPN circuits were almost unknown, although they were vaguely implicated in emotion. Because of this lack of knowledge, I thought that the habenula would be more interesting than motor neurons, and decided to shift the focus of my study to the habenula. We already discovered in adult zebrafish that the two parallel pathways from the dorsal habenula to the interpeduncular nucleus (IPN) function antagonistically for zebrafish to behave either as a winner or a loser in the social conflict over dominance. We recently discovered that the same winner pathway is also essential for fish to make a decision whether to turn left or right to gain food. These observations have led us to the hypothesis that the IPN output in the winner pathway may encode the prediction of the favorable interoceptive states such as body flexion for proper targeting of the object. With regard to the loser’s pathway, we have recently found that the activities of the 5HT neurons in the median raphe, the terminus of this pathway, are involved in the evaluation of the level of danger or safety of the surrounding environment. We also have succeeded in recapitulating this social fight into the virtual reality space and observing the activities of neurons in the involved pathways.
Concomitantly, a report was published that the zebrafish telencephalon has an evolutionarily conserved basic structure similar to the mammalian telencephalon, which was against the prevailing common sense that fish lacks the structure of the brain necessary for adaptive intelligence such as the cortex and the basal ganglia. This has led me to think that we can address how the cortico-basal neural circuits regulate the decision-making process both from the neural circuit and the computational aspects by taking advantage of much smaller zebrafish telencephalon than that of mammals. Although it has taken us a significantly long time and hard work, I think that my initial idea has finally been materialized as a unique experimental system that can be used for addressing versatile and broad fundamental questions of decision-making. In this virtual reality system where adult zebrafish make decisions as to Go or No-Go under given environments, observation of the neural activities in the dorsal telencephalon equivalent to the mammalian cortex suggested that fish behave so that the activities of the neurons encoding the prediction error between the favorable situation expected by the internal model of the brain and the real situation is minimized, implicating the fish brain’s capacity of forming the internal model for predictive coding and its use for behavioral optimization.
By taking full advantage of our ability to generate various genetically modified strains and virusmediated neural tracing and manipulations combined with our unique in vivo neural imaging systems under the closed-loop virtual reality condition, we plan to address these subjects by identifying the underlying neural circuits and their functions.
Based on the knowledge gained by studying the adult zebrafish brain, we would also like to prove either by ourselves or by collaboration with other experts that the same principles work in mammals including humans.
A. Habenula as the experience-dependent controlling switchboard of behavior and attention in social conflict and learning
​
We found that the two adjacent neural pathways, i.e. one from the lateral subnucleus of the dorsal habenula (dHbL) to the dorsal part of the interpeduncular nucleus (dIPN) and the other from the medial subnucleus of the dorsal habenula (dHbM) to the ventral part of the interpeduncular nucleus (vIPN), play the antagonistic roles in the regulation of the competitiveness in the social conflict over dominance and submission (Fig. 1, 2)[ref. 2,4,5,6,7]. The transgenic inactivation of the neural transmission from the dHbL to dIPN makes zebrafish prone to lose, while the inactivation of the dHbM-vIPN pathway makes fish invincible in fight. We have discovered that the dHbL-dIPN-(dorsal tegmental area, DTA) pathway is potentiated in the winner’s brain, while the dHbM-vIPN-(median raphe, MR) pathway is potentiated in the loser’s brain. The recent research in mice by others has indicated that interbrain synchrony could reflect the social hierarchy, i.e., neurons in the prefrontal cortex in winners respond better to their own behavior compared to the loser animals, while the losers’ neurons respond more to the opponent winners’ behavior.
Navigation behavior for appetitive reward, such as foraging, is excellent to study how animals are engaged in attending to specific information to achieve favorable outcomes. The choice to use different navigation strategies, i.e. whether to depend on landmarks or dead-reckoning, allows to conceptually divide information in terms of differences of the source. In landmark navigation, animals perceive the external cues through primary sensory modalities, such as olfaction, hearing, and vision. In contrast, dead-reckoning relies on an integrated internal signal such as vestibular and proprioceptive signaling. We designed an operant learning paradigm for obtaining appetitive reward (food) to monitor the learning and decisionmaking process of zebrafish by adopting either the internal-directional information (left or right turn) or the external-cued information (turning toward the red- or blue-colored goal) with an automatic training system using the plus maze (Fig. 3A)[ref. 4,5]. We found that the zebrafish can achieve the training paradigm by exploiting both internal-directional information, e.g. left or right, and external-cued information, e.g. red or blue goal color. Quite intriguingly, we found that the fish with defective neural transmission from the lateral subnucleus of the dorsal habenula (dHbL) to the dorsal part of the interpeduncular nucleus (dIPN) by genetic manipulation showed the specific defect in learning by exploiting the internal-directional information, while keeping its capacity to learn by using external-cued information unaffected. This result demonstrated that the dHbL is indispensable for utilization of internal-directional information but not for external-cued information in the zebrafish operant learning behavior (Fig. 3B).
​
Considering that the dHbL-d/iIPN-DTA pathway plays essential roles both in facilitating fish to behave as winners and in decision-making depending on the internal-directional information (left or right), the winning experience in dyadic fighting could synergistically induce a weight shift of information selection from the other-directed to self-centered selection, concomitantly with the brain state changing towards being more egocentric (Fig. 4)[ref. 4].
These results have led us to the working hypothesis that the two parallel dHb-IPN pathways are involved in controlling internal (self-centered)/external (other-directed) selection of information, suggesting that they could act as the integrated switchboard for concertedly controlling zebrafish to behave either as a winner with self-centered (idiothetic) attention or a loser with others-oriented (allothetic) attention (Fig. 4).


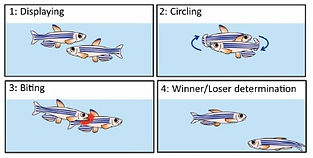
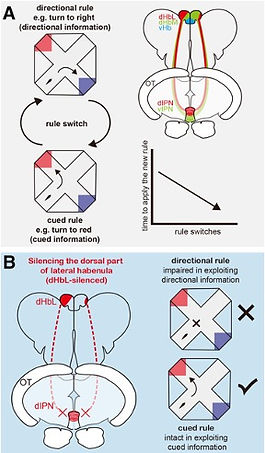

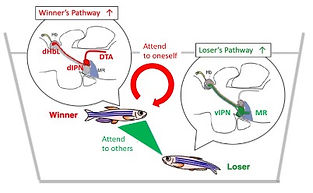

B. zebrafish brain as a simple mini-brain model of higher vertebrate brain to study the evolutionarily conserved mechanism of decision-making
The capacity to predict the desirable future and to plan the proper behavioral strategy to realize it has been regarded as the inherent function of the higher vertebrate brain in decision-making. However, adult zebrafish has recently drawn attention as a model animal for the study of decision-making due to its capability of various adaptive behaviors and the conservation of the basic telencephalic structure, which contributes to decision-making. To analyze the neural activities of the fish telencephalon during learning of active avoidance, we have established the closed-loop virtual reality system for the head-tethered adult zebrafish with the 2-photon calcium imaging system (Fig. 5)[ref. 1,3,8]. The adult zebrafish harboring G-CaMP7 in the excitatory neurons were trained to perform visual-based active and passive avoidance tasks, i.e., fish must escape when the surrounding landscape turns blue and stay when the landscape turns red, and simultaneously, the neural activities were imaged at the cellular level. The Non-negative Matrix Factorization analysis revealed two ensembles that attribute rules to the colors of the surrounding landscape, i.e. blue is dangerous and red is safe (Fig. 6). In addition, we observed another ensemble in one-third of the successfully learned fish whose activity was suppressed by the perception of the backward movement of the landscape as seen when fish was successfully swimming forward to escape from the blue to the red compartment (Fig. 6). This result suggested that the third ensemble encoded the error from the prediction of favorable perceptual state to achieve the successful active avoidance, i.e. perceiving the visual flow of backward movement of the landscape. To test this, we artificially enhanced this prediction error by introducing the open-loop condition in which fish could not swim forward and could not reach the goal, however hard fish beat the tail. In this condition, this ensemble was activated. Quite intriguingly, zebrafish with both the rule-encoding ensembles and the ensemble encoding the sensory-flow prediction error swam more efficiently toward the goal without taking rest than the fish with only the rule-encoding ensembles which swam toward the goal intermittently, suggesting that fish with the error-encoding ensemble selected the behavior so that this error would be minimized (Fig. 7). Our observation suggested that zebrafish can make decision according to the so-called “Active Inference”, in which animals adopt their behaviors to minimize the prediction error (surprise or more precisely “free energy”) between the current real state and the desirable predicted state. Our results have strongly demonstrated the usefulness of the zebrafish brain as a simple mini-brain model of the higher vertebrate brain to study the evolutionarily conserved mechanism of decision-making based on the internal model.
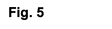
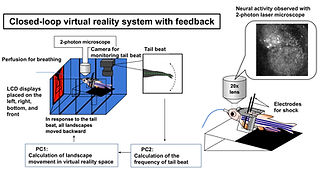
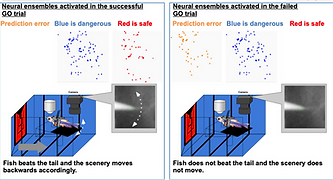
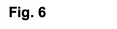
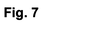
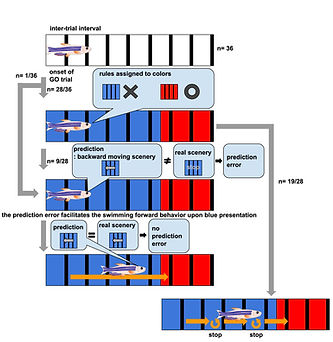
----------------------------------------------------------------------------------------------------------------------------------------
Hitoshi Okamoto M.D., Ph.D
​
POSITION TITLE:
RIKEN Honorary Scientist, Visiting Senior Scientist, RIKEN Center for Brain Science
Visiting Senior Scientist, Waseda University and Institute of Neuropsychiatry
ADDRESS: 2-1 Hirosawa, Wako, Saitama, 351-0198 Japan,
TEL: +81-5035026094
E-MAIL: hitoshi.okamoto@riken.jp
​​
CV
1983 M.D. Tokyo Univ.
1988 Ph.D. Tokyo Univ. (advisor, Prof. Yoshiki Hotta)
1988~1991 Postdoctoral Research Fellow, Univ. of Michigan, Ann Arbor
(advisor, Prof. John Y. Kuwada)
1991~1993 Assistant Professor, National Institute for Basic Biology
1993~1997 Lecturer and Associate Professor, Dept. of Physiology,
Keio Univ., School of Med.
1997~2018, Laboratory Head, Lab. for Developmental Gene Regulation, BSI
2004~2008, Group Director, Neural Growth and Regeneration Research Group, BSI
2008~2018, Deputy Director of BSI,
2009~2018, Senior Team Leader, BSI
2018~2024(March) Team Leader, RIKEN Center for Brain Science (CBS)
Lab. for Neural Circuit Dynamics of Decision Making
Adjunct Professor of Waseda Univ., Keio Tokyo Univ.
2024(April) ~ Present
RIKEN Honorary Scientist, Visiting Senior Scientist
RIKEN Center for Brain Science
Visiting Senior Scientist, Waseda University and Institute of Neuropsychiatry
Project Leader of the National Bioresource Project (Zebrafish) (NBRP-Z)
Other Activities
Board Member of Japanese Society for Neuroscience
Associate Member of Science Council of Japan
Scientific Advisory Board member, Max Planck Institute of Animal Intelligence
(Past)
Member of the Future Planning Committee of the Union of Brain Science Association in Japan
Chair of the International Brain Research Organization (IBRO) Asia-Pacific Regional Committee(APRC)
​
Colleagues
[List of Selected Papers]
The list of my all works:
https://scholar.google.com/citations?hl=en&user=4RW7wK4AAAAJ&view_op=list_works
​
1.Tanimoto Y, Kakinuma H, Aoki R, Shiraki T, Higashijima S, Okamoto H (2024) Transgenic tools targeting the basal ganglia reveal both evolutionary conservation and specialization of neural circuits in zebrafish. Cell Reports, 3(3):113916. doi: 10.1016/j.celrep.2024.113916.
​
2.Kinoshita M, Okamoto H. (2023) Acetylcholine potentiates glutamate transmission from the habenula to the interpeduncular nucleus in losers of social conflict. Curr Biol. 33(11):2121-2135.e4. doi: 10.1016/j.cub.2023.03.087. PMID: 37105168
​
3.Torigoe M, Islam T, Kakinuma H, Fung CCA, Isomura T, Shimazaki H, Aoki T, Fukai T, Okamoto H. (2022) Zebrafish capable of generating future state prediction error show improved active avoidance behavior in virtual reality. Nat Commun. 12(1):5712. doi: 10.1038/s41467-021-26010-7.PMID: 34588436
​
4.Okamoto H, Cherng BW, Nakajo H, Chou MY, Kinoshita M.(2021) Habenula as the experience-dependent controlling switchboard of behavior and attention in social conflict and learning. Curr Opin Neurobiol. 68:36-43.
​
5.Cherng BW, Islam T, Torigoe M, Tsuboi T, Okamoto H. (2020) The Dorsal Lateral Habenula-Interpeduncular Nucleus Pathway Is Essential for Left-Right-Dependent Decision Making in Zebrafish. Cell Rep. 32(11):108143. doi: 10.1016/j.celrep.2020.108143. PMID: 32937118
​
6.Nakajo H, Chou MY, Kinoshita M, Appelbaum L, Shimazaki H, Tsuboi T, Okamoto H. (2020) Hunger Potentiates the Habenular Winner Pathway for Social Conflict by Orexin-Promoted Biased Alternative Splicing of the AMPA Receptor Gene. Cell Rep. 31(12):107790. doi: 10.1016/j.celrep.2020.107790.PMID: 32579920.
​
7.Chou M, Amo R, Kinoshita M, Cherng B, Shimazaki H, Agetsuma M, Shiraki T, Aoki T, Takahoko M, Yamazaki M, Higashijima S, Okamoto H (2016) Social conflict resolution regulated by two dorsal habenular subregions in zebrafish. Science 352:87-90
​
8.Amo, R., Fredes, F., Kinoshita, M., Aoki, R., Aizawa, H., Agetsuma, M., Aoki, T., Shiraki, T., Kakinuma, H., Matsuda, M., Yamazaki, M., Takahoko, M., Tsuboi, T., Higashijima, S., Miyasaka, N., Koide, T., Yabuki, Y., Yoshihara, Y., Fukai, T., Okamoto, H. (2014) The habenulo-raphe serotonergic circuit encodes an aversive expectation value essential for adaptive active avoidance of danger. Neuron, 84:1034-1048.
9.Aoki, T., Kinoshita, M., Aoki, R., Agetsuma, M., Aizawa, H., Yamazaki, M., Takahoko, M., Amo, R., Arata, A., Higashijima, S., Tsuboi, T., Okamoto, H. (2013) Imaging of Neural Ensemble for the Retrieval of a Learned Behavioral Program. Neuron 78:881-94.
​
10.Ohata, S., Aoki, R., Kinoshita, S., Yamaguchi, M., Tsuruoka-Kinoshita, S., Tanaka, H., Wada, H., Watabe, S., Tsuboi, T., Masai, I. Okamoto, H. (2011) Dual Roles of Notch in Regulation of Apically Restricted Mitosis and Apicobasal Polarity of Neuroepithelial Cells. Neuron, 69:215-230.
​
11.Agetsuma, M., Aizawa, H., Aoki, T., Nakayama, R., Takahoko, M., Goto, M., Sassa, T., Amo, R., Shiraki, T., Kawakami, K., Hosoya, T., Higashijima, S., Okamoto, H. (2010) The habenula is crucial for experience-dependent modification of fear responses in zebrafish. Nature Neurosci., 13:1354-1356.
​
12.Aizawa, H., Goto, M., Okamoto, H. (2007) Temporally regulated asymmetric neurogenesis causes left-right difference in the zebrafish habenular structures Devel. Cell, 12:87-98.
 
13.Wada, H., Tanaka, H., Nakayama, S., Iwasaki, M., and Okamoto, H. (2006). Frizzled3a and Celsr2 regulate neuroepithelial exclusion of migrating facial motor neurons in the zebrafish hindbrain. Development, 133:4749-59.
​
14.Masai, I., Yamaguchi, M., Tonou-Fujimori, N., Komori, A., and Okamoto, H. (2005). Requirement of Hedgehog signalling for the cell-cycle exit of retinal progenitor cells in zebrafish. Development, 132:1539-1553.
​
15.Aizawa, H., Bianco, I.H., Hamaoka, T., Miyashita, T., Uemura, O., Concha, M.L., Russell, C., Wilson, S.W., and Okamoto, H., (2005) Laterotopic Representation of Left-Right Information onto the Dorso-Ventral Axis of a Zebrafish Midbrain Target Nucleus, Curr. Biol., 15: 238-243.
​
16.Wada, H., Iwasaki, M., Sato, T., Masai, I., Nishiwaki, Y., Tanaka, H., Sato, A., Nojima, Y., and Okamoto, H. (2005). Dual roles of zygotic and maternal Scribble1 in neural migration and convergent extension movements in zebrafish embryos. Development, 132:2273-2285.
​
17.Ando, H., Furuta, T., Tsien, R. Y. and Okamoto, H. (2001) Photo-mediated gene activation using caged RNA/DNA in zebrafish embryos. Nature Genetics, 28: 317 – 325.
​
18.Segawa, H., Miyashita, T., Hirate Y., Higashijima, S., Chino, N., Uyemura, K., Kikuchi, Y. and Okamoto, H. (2001) Functional repression of Islet-2 by disruption of the heteromeric complex with Ldb impairs peripheral axonal outgrowth by the primary sensory and motor neurons in embryonic zebrafish. Neuron, 30: 423-436.
​
19.Higashijima, S., Hotta, Y., and Okamoto, H. (2000) Visualization of cranial motor neurons in live transgenic zebrafish expressing GFP under the control of the Islet-1 promoter/enhancer. J. Neurosci., 20: 206-218.
​
20.Kikuchi, Y., Segawa, H., Tokumoto, M., Tsubokawa, T., Hotta, Y., Uyemura, K., and Okamoto, H.(1997). Ocular and cerebellar defects in zebrafish induced by overexpression of the LIM domain of the Islet-3 LIM/homeodomain protein. Neuron, 18:369-382.
​
21.Tokumoto, M., Gong,Z., Tsubokawa, T., Hew,C., Hotta, Y. Uyemura,K., and Okamoto, H. (1995). Molecular heterogeneity among primary moto-neurons and within myotomes revealed by differential mRNA expression of novel Isl-1 homologs in embryonic zebrafish. Dev. Biol., 171:578-589.
​
22.Inoue, A., Takahashi, M., Hatta, K., Hotta, Y., and Okamoto, H. (1994). Developmental regulation of Isl-1 mRNA expression during neuronal differentiation in embryonic zebrafish. Dev. Dynam., 199:1-11.
​